Quantum science and technology: highlights of 2023

It’s been another banner year for quantum science and technology, with academic research groups and tech firms celebrating significant achievements in quantum computing, quantum communications and quantum metrology as well as fundamental quantum science. Three of these advances – a quantum repeater that transmits quantum information over a distance of 50 km; a double-slit experiment in time; and a simulation of an expanding universe in a Bose-Einstein condensate – appeared in our list of the year’s top 10 breakthroughs, but with so many exciting things going on, we can’t resist celebrating a few others. Here, in no particular order, are some highlights.
Joining the hardware dots
Some innovations hit the headlines right away. Others lay the groundwork for future breakthroughs. In May, Johannes Fink and colleagues at the Institute for Science and Technology Austria claimed a place in the second group by demonstrating a protocol for entangling microwave and optical photons. This is important because the superconducting circuits that make up many of today’s most advanced quantum computers operate at microwave frequencies, but the fibres and other equipment used to send information over long distances work at optical frequencies. If we want to build a network of many quantum computers and make them talk to each other, we will therefore need strong, reliably quantum connections between these two frequencies.
Now that Fink and his team have shown that such connections are possible, prospects for quantum networks based on superconducting qubits look rosier, though the protocol still needs refining. As one independent expert observed, “We should not think that this makes everything easy now – it’s just the beginning, but that doesn’t take away from the quality of the experiment.â€

A similarly slow-burn advance occurred in August when researchers in John Bowers’ group at the University of California, Santa Barbara, put a laser and a photonic waveguide on the same chip for the first time. Integrated photonic systems like these will be crucial to scaling up quantum computers based on trapped ions or atoms, but lasers and waveguides haven’t always played well together. Specifically, when light from a laser enters a waveguide, some of it gets reflected, and if this reflected light gets back to the laser, the laser’s output becomes unstable. By designing a chip that avoids these unwanted interactions, Bowers and colleagues made the job of future quantum hardware designers much easier.
Milestones in quantum metrology
In the year the first commercial optical atomic clock went on sale, quantum metrologists also notched up an achievement at the other end of the technology readiness scale. Just as optical clocks are more precise than their microwave-frequency predecessors, clocks that “tick†every time an atom’s nucleus undergoes an energy transition would be more precise still. They might even be precise enough to catch fundamental constants in the act of fluctuating on very short time scales, which would violate the Standard Model of particle physics.
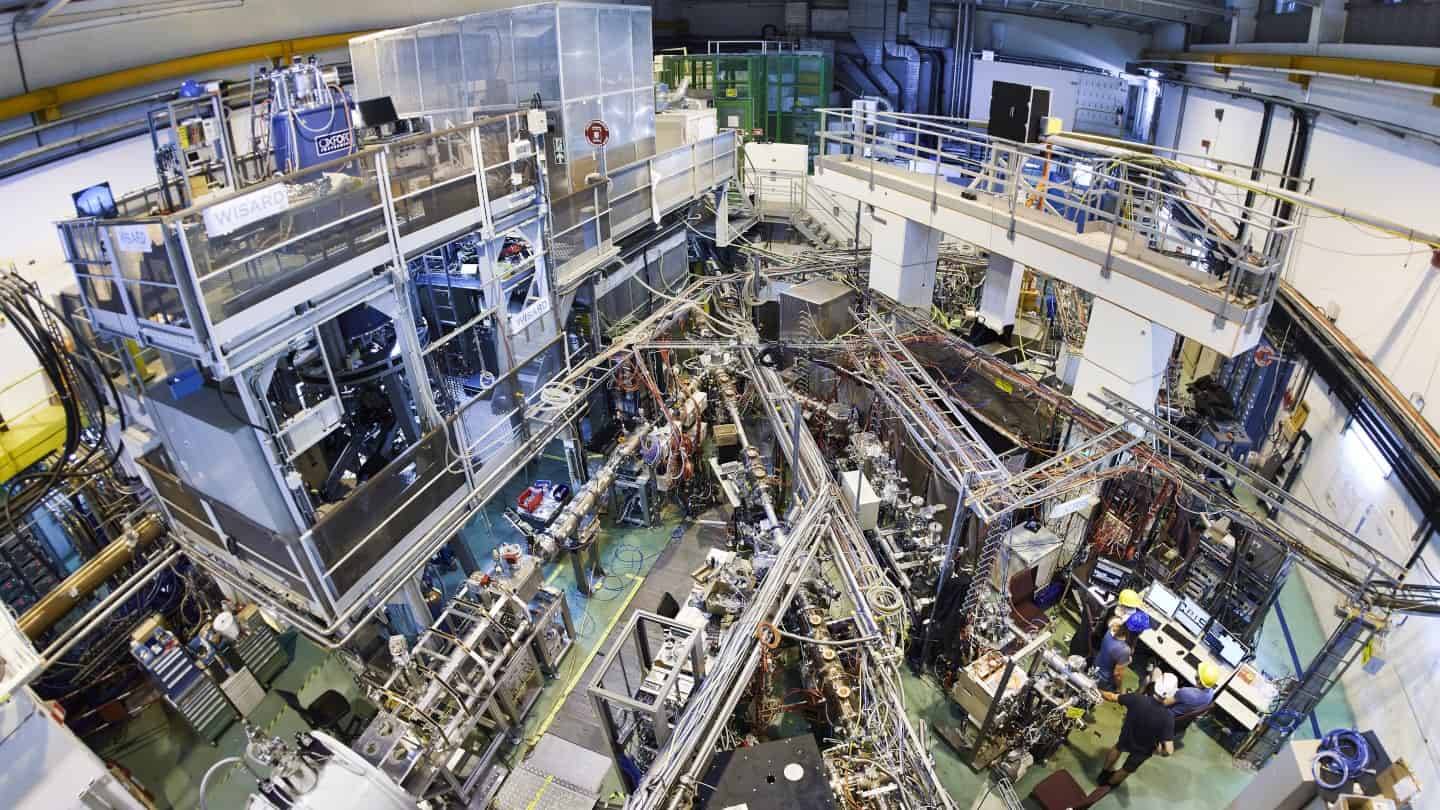
The problem is that no-one knows the frequencies of these nuclear transitions well enough to drive them with a laser. In June, though, physicists at CERN got closer to finding out when they detected a photon emitted by a thorium-229 ion as it returned to its nuclear ground state. Though much work remains to be done, the result is nonetheless a step towards the next generation of ultra-precise timekeeping.
Meanwhile, physicists at the University of Colorado, Boulder, US, put down a marker in their quest to measure the electron electric dipole moment (eEDM) to ever-greater precision. A non-zero value of this quantity would violate the Standard Model, and in August, a team led by Jun Ye and Eric Cornell announced that the eEDM must be less than 4.1 x 10-30 e cm, with an uncertainty of 2.1×10-30 – a precision equivalent to measuring the Earth to within the dimensions of a virus.
The emergence of effective quantum error correction

Errors are the bane of quantum computers, and demonstrating ways to correct them is a major goal of quantum computing research. In 2023, these efforts started to pay off. In February, researchers at Google Quantum AI announced that they had suppressed errors in their superconducting-qubit device by implementing a surface code. This type of quantum error-correcting code encodes a logical (that is, error-corrected) qubit in the joint entangled state of many physical qubits. The following month, a team at Yale University in the US demonstrated a different approach to the same problem, using a qubit encoding called a GKP code to suppress errors with the help of additional information embedded in superconducting transmon qubits.
Top 10 Breakthroughs of 2023: we explore this year’s best physics research
Arguably the year’s most impressive error-correction result, however, came just a few weeks ago, when Mikhail Lukin and colleagues at Harvard University, QuEra Computing, the Massachusetts Institute of Technology and the NIST/University of Maryland Joint Center for Quantum Information and Computer Science reported that they had created an array of 48 logical qubits using neutral atoms.
Even before this announcement, 2023 was looking like a breakout year for neutral-atom quantum computers, which are having a moment after a long period of trailing behind devices that use superconducting circuits or trapped ions as qubits. Will 2024 be the year they leap ahead? Or will their rivals find new advantages to exploit? Watch this space!
The best of the rest
Finally, a few of 2023’s quantum achievements stand out for their sheer ingenuity. This year saw the first observation of quantum superchemistry, which occurs when chemical reactions speed up because the reacting molecules are all in the same quantum state. It also marked the first time anyone had spotted quantum entanglement in top quarks, which have a lifetime of just 10-25 seconds. The most ingenious quantum result of the year, though, is surely the demonstration of an engine that runs on the energy difference between bosons and fermions. As an example of the links between classical and quantum physics, it could hardly be better.
Physics World‘s coverage of the Breakthrough of the Year is supported by Reports on Progress in Physics, which offers unparalleled visibility for your ground-breaking research.